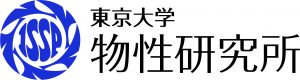
ISSP workshop/ 物性研究所短期研究会
“Frontier of scanning probe microscopy and related nano science”
「機能的走査プローブ顕微鏡の新展開」
Date, Time (JST):
March 30, 2022 (Wed.) 13:30-20:30
March 31, 2022 (Thu.) 9:00-18:00
Registration deadline (JST):
Poster presentation: March 23, 2022 (Wed.)
Extended → March 28, 2022 (Mon.)
Poster student award will be given.
優秀な学生講演には賞が贈られます。
Audience: March 31, 2022 (Thu.)
Registration fee:
Free/無料
Venue:
Online/ オンライン開催
Language:
Hybrid of English and Japanese (depending on the session)
セッション毎の日本語・英語のハイブリッド
Congratulations!
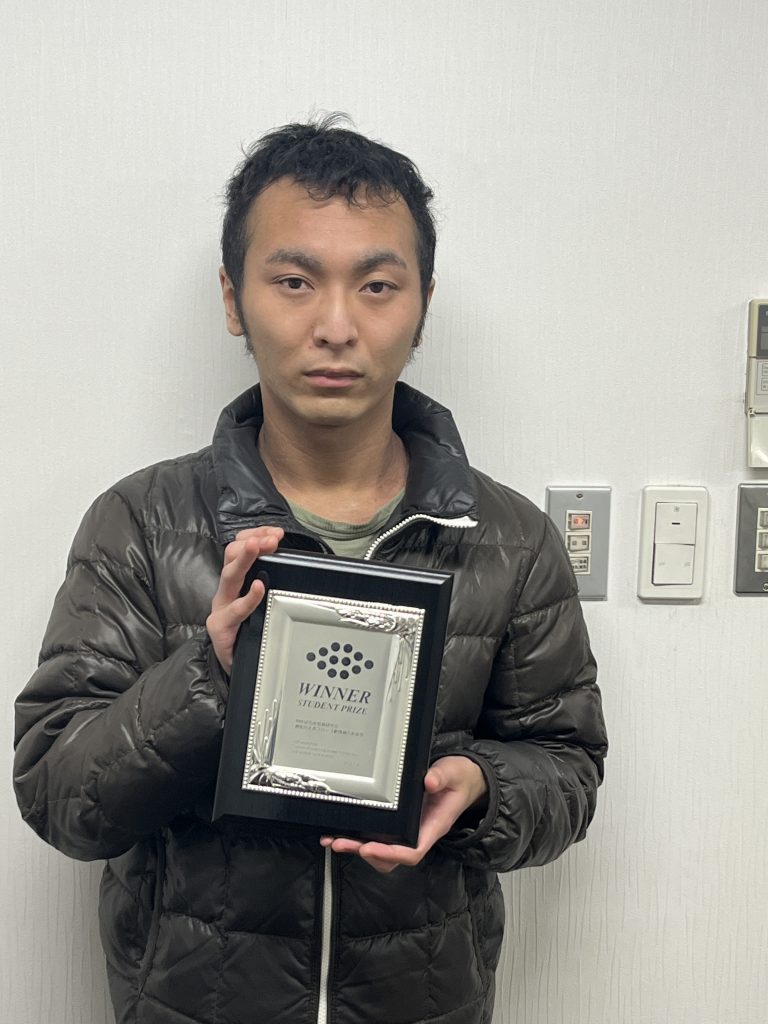
ポスター講演における学生賞を大阪大学の三坂朝基さんが受賞しました!
おめでとうございます!
Program
The assigned time is 30 minutes for your presentation + 10 minutes for discussion.
講演は、30分発表+10分討論を予定しています。
March 30, 2022 (Wed.)
13:30- 所長挨拶 森初果
Session1(Japanese/日本語)
Chair:Yukio Hasegawa (長谷川幸雄)
13:40-14:20 Kohei Yamasue (Tohoku Univ.)/山末 耕平 (東北大)
「時間分解SNDMを用いた半導体のナノスケール評価に関する最近の展開 」
[1] Y. Cho, A. Kirihara, and T. Saeki, Rev. Sci. Instrum. 67, 2297-2303(1996).
[2] Y. Cho, Scanning Nonlinear Dielectric Microscopy: Investigation of Ferroelectric, Dielectric, and Semi-conductor Materials and Devices, Elsevier, ISBN 9780128172469 (2020).
[3] Y. Cho, Jpn. J. Appl. Phys. 56, 100101 (2017).
[4] Y. Yamagishi and Y. Cho, Appl. Phys. Lett. 111, 163103 (2017).
[5] K. Yamasue and Y. Cho, in 2021 IEEE International Symposium on the Physical and Failure Analysis of Integrated Circuits (IPFA) (2021).
[6] K. Suzuki, K. Yamasue, and Y. Cho, in 2019 IEEE International Integrated Reliability Workshop (IIRW) (2019).
14:20-15:00 Junsuke Yamanishi (IMS)/山西 絢介 (分子研)
「光誘起力顕微鏡に関する最近の動向 」
[1] I. Rajapaksa et al., Appl. Phys. Lett. 97, 073121 (2010).
[2] J. Jahng et al., Acc. Chem. Res. 48, 2671 (2015).
[3] I. Rajapaksa et al., Appl. Phys. Lett. 99, 161103 (2011).
[4] J. Jahng et al., Appl. Phys. Lett. 106, 083113 (2015).
[5] J. Jahng et al., Anal. Chem. 90, 11054-11061 (2018).
[6] J. Yamanishi et al., Phys. Rev. Applied 9, 024031 (2018).
[7] J. Yamanishi et al., Nat. Commun. 12, 3865 (2021).
15:00-15:40 Yusuke Kajihara(Univ. Tokyo)/梶原 優介 (東京大)
「ナノサーモメトリーを実現するパッシブ近接場顕微鏡」
[1] K. Joulain, J.P. Mulet, F. Marquier, R. Carminati, and J.J. Greffet, Surf. Sci. Rep. 57, 59 (2005).
[2] S. Komiyama, IEEE J. Sel. Top. Quantum Electron. 17, 54 (2011).
[3] Y. Kajihara, K. Kosaka, and S. Komiyama, Opt. Express, 19, 8, 7695 (2011).
[4] K-T. Lin, H. Nema, Q. Weng, S. Kim, K. Sugawara, T. Otsuji, S. Komiyama, and Y. Kajihara, Applied Physics Express, 13, 9 , (2020) 96501.
[5] R. Sakuma, K-T. Lin, S. Kim, F. Kimura, and Y. Kajihara, Rev. Sci. Instrum., 93 (2022) 13704.
Break/休憩
Session2(English)
Chair: Tetsuo Hanaguri (花栗哲郎)
16:00-16:40 Peter Liljeroth (Aalto University, Finland)
「Designer quantum states in van der Waals heterostructures」
[1] S. Kezilebieke et al. Topological superconductivity in a van der Waals heterostructure, Nature 588, 424-428 (2020).
[2] S. Kezilebieke et al. Moiré-enabled topological superconductivity, Nano Lett. 22, 328 (2022).
[3] V. Vaňo et al. Artificial heavy fermions in a van der Waals heterostructure, Nature 599, 582-586 (2021).
16:45-17:25 Eli Zeldov (Weizmann Institute of Science, Israel)
「Nanoscale thermal imaging: Glimpse into dissipation in quantum systems down to atomic scale」
[1] D. Halbertal, J. Cuppens, M. Ben Shalom, L. Embon, N. Shadmi, Y. Anahory, H. R. Naren, J. Sarkar, A. Uri, Y. Ronen, Y. Myasoedov, L. S. Levitov, E. Joselevich, A. K. Geim, and E. Zeldov, Nature 539, 407 (2016).
[2] D. Halbertal, M. Ben Shalom, A. Uri, K. Bagani, A.Y. Meltzer, I. Marcus, Y. Myasoedov, J. Birkbeck, L.S. Levitov, A.K. Geim, and E. Zeldov, Science 358, 1303 (2017).
[3] A. Marguerite, J. Birkbeck, A. Aharon-Steinberg, D. Halbertal, K. Bagani, I. Marcus, Y. Myasoedov, A.K. Geim, D.J. Perello, and E. Zeldov, Nature 575, 628 (2019).
17:30-18:10 Milan P. Allan (Leiden University, Netherlands)
「Electron pairs without superconductivity in a disordered superconductor」
[1] KM Bastiaans et al., RSI 89, 093709 (2018)
[2] KM Bastiaans, D. Cho et al., Nature Physics 14, 1183 (2018)
[3] KM Bastiaans et al., Phys. Rev. B 100, 104506 (2019)
[4] KM Bastiaans, et al. Science 374, 608 (2021)
Allanlab.org
18:10-20:30 poster session/ポスター講演
March 31, 2022 (Thu.)
Session3 (English)
Chair:Toshu An (安東秀)
9:00-9:40 Jiaqi Zhang (Japan Advanced Institute of Science and Technology, Japan)
「atomic scale mechanics studied by in-situ transmission electron microscopy with a quartz length-extension resonator」
Group Homepage
9:45-10:25 Soohyon Phark (Institute for basic science, Korea)
「Atomic Scale Electron Spin Resonance: towards Electron Spin Qubits on a Surface」
[1] K. Yang et al. Science 366, 509-512 (2019).
[2] P. Willke et al. ACS Nano 15, 17959-17965 (2021).
[3] J. L. Lado et al. Phys. Rev. B 96, 205420 (2017).
[4] K. Yang et al. Phys. Rev. Lett. 122, 227203 (2019).
[5] J. R. Galvez et al. Phys. Rev. B 100, 035411 (2019).
[6] S. Phark et al. arXiv:2108.09880 (2021).
Break/休憩
Session4(日本語)
座長:Masahiro Haze (土師将裕)
10:50-11:30 Yuta Kainuma (JAIST)/貝沼 雄太(北陸先端大)
「走査ダイヤモンドNV中心プローブの開発と磁気イメージング 」
1 M.S. Grinolds, S. Hong, P. Maletinsky, L. Luan, M.D. Lukin, R.L. Walsworth, and A. Yacoby, Nat. Phys. 9, 215 (2013).
2 A. Ariyaratne, D. Bluvstein, B.A. Myers, and A.C.B. Jayich, Nat. Commun. 9, 2406 (2018).
3 D. Kikuchi, D. Prananto, K. Hayashi, A. Laraoui, N. Mizuochi, M. Hatano, E. Saitoh, Y. Kim, C.A. Meriles, and T. An, Appl. Phys. Express 10, 103004 (2017).
4 D. Prananto, Y. Kainuma, K. Hayashi, N. Mizuochi, K.I. Uchida, and T. An, Phys. Rev. Appl. 16, 064058 (2021).
5 T.X. Zhou, J.J. Carmiggelt, L.M. Gächter, I. Esterlis, D. Sels, R.J. Stöhr, C. Du, D. Fernandez, J.F. Rodriguez-Nieva, F. Büttner, E. Demler, and A. Yacoby, Proc. Natl. Acad. Sci. U. S. A. 118, e2019473118 (2021).
6 Y. Kainuma, K. Hayashi, C. Tachioka, M. Ito, T. Makino, N. Mizuochi, and T. An, J. Appl. Phys. 130, 243903 (2021).
研究室HP 研究室Twitter
11:35-12:15 Takashi Kumagai (IMS)/熊谷 崇(分子研)
「原子スケールの極微分光」
1. B. Cirera et al. Charge Transfer-Mediated Dramatic Enhancement of Raman Scattering upon Molecular Point Contact Formation. Nano Letters
2. S. Liu et al. Anti-Stokes Light Scattering Mediated by Electron Transfer Across a Biased Plasmonic Nanojunction. ACS Photonics 8, 2610–2617 (2021).
3. S. Liu et al. Atomic Point Contact Raman Spectroscopy of a Si (111)-7× 7 Surface. Nano Letters 21, 4057–4061 (2021).
4. S. Liu et al. Dramatic Enhancement of Tip-Enhanced Raman Scattering Mediated by Atomic Point Contact Formation. Nano Letters 20, 5879–5884 (2020).
5. S. Liu et al. Resolving the Correlation between Tip-Enhanced Resonance Raman Scattering and Local Electronic States with 1 nm Resolution, Nano Letters 19, 5725–5731 (2019).
6. H. Böckmann et al. Near-Field Manipulation in a Scanning Tunneling Microscope Junction with Plasmonic Fabry-Pérot Tips. Nano Letters 19, 3597–3602 (2019).
7. H. Böckmann et al. Near-Field Spectral Response of Optically Excited Scanning Tunneling Microscope Junctions Probed by Single-Molecule Action Spectroscopy. J. Chem. Phys. Lett. 10, 2068–2074 (2019).
8. H. Böckmann et al. Near-Field Enhanced Photochemistry of Single Molecules in a Scanning Tunneling Microscope Junction. Nano Letters 18, 152–157 (2018).
Break/休憩
Session4(日本語)
座長:Yoshiaki Sugimoto (杉本宜昭)
13:30-14:10 Takeshi Fukuma (Kanazawa Univ.)/福間 剛士(金沢大)
「AFMによる3次元自己組織化構造の内部観察」
1. Penedo, M.; Miyazawa, K.; Okano, N.; Furusho, H.; Ichikawa, T.; Alam Mohammad, S.; Miyata, K.; Nakamura, C.; Fukuma, T., Visualizing intracellular nanostructures of living cells by nanoendoscopy-AFM. Sci. Adv. 2021, 7 (52), eabj4990.
2. Fukuma, T.; Garcia, R., Atomic- and Molecular-Resolution Mapping of Solid-Liquid Interfaces by 3D Atomic Force Microscopy. ACS Nano 2018, 12 (12), 11785-11797.
3. Asakawa, H.; Yoshioka, S.; Nishimura, K.; Fukuma, T., Spatial Distribution of Lipid Headgroups and Water Molecules at Membrane/Water Interfaces Visualized by Three-Dimensional Scanning Force Microscopy. ACS Nano 2012, 6 (10), 9013-9020.
研究室HP 研究所HP
14:15-14:55 Norio Okabayashi (Kanazawa Univ.)/岡林 則夫(金沢大)
「外場による単一分子の構造変化とエネルギー散逸」
[1] F. J. Giessibl, Rev. Sci. Instrum. 90, 011101 (2019)
[2] N. Okabayashi et al., PRB 93, 165415 (2016).
[3] N. Okabayashi, A. Peronio, M. Paulsson, T. Arai, and F. J. Giessibl, PNAS. 115, 4571 (2018).
[4] A. Peronio, N. Okabayashi, F. Griesbeck, F. Giessibl, Rev. Sci. Instrum. 90, 123104 (2019).
[5] N. Okabayashi, T. Frederiksen, A. Liebig, F. J. Giessibl. arXiv:2112.12286
研究者HP
15:00-15:40 Hiroyuki Koshida (Univ. Tokyo)/越田 裕之(東京大)
「金属表面におけるNOの吸着状態と磁性」
[1] A. Yoshimori. Surf. Sci., 342(1-3):L1101–L1103, 1995.
[2] R. Requist et al. Proc. Natl. Acad. Sci. USA, 111(1):69–74, 2014.
[3] H. Koshida et al. Phys. Rev. B 103.15 (2021): 155412.
Break/休憩
Session5(日本語)
座長: Masahiro Haze (土師将裕)
16:00-16:40 Koichiro Ienaga (Tokyo Tech.)/家永 紘一郎(東工大)
「単層近藤格子CePt2/Pt(111)の成長と電子状態」
[1] H. Shishido, T. Shibauchi, K. Yasu, T. Kato, H. Kontani, T. Terashima, Y. Matsuda, Science 327, 980 (2010).
[2] J. Kemmer, C. Praetorius, A. Krönlein, P.-J. Hsu, K. Fauth, and M. Bode, Phys. Rev. B 90, 195401 (2014).
[3] M. Klein et al., Phys. Rev. Lett. 106, 186407 (2011).
[4] K. Ienaga, S. Kim, T. Miyamachi, and F. Komori, Phys. Rev. B 104, 165419 (2021).
研究室HP
16:45-17:25 Tomoya Asaba (Kyoto Univ.)/浅場 智也(京都大)
「 チューリング不安定性による原子スケールワイヤおよびジャンクションの作製 」
17:30- Closing
Registration
締めきりました
Sponsored by:
Institute for Solid State Physics, University of Tokyo
東京大学物性研究所
Co-sponsored by:
Scanning probe microscopy committee in the Japan Society of Vacuum and Surface Science
日本表面真空学会走査プローブ顕微鏡研究部会
Committee members:
Toshu An (JAIST)/安東秀(北陸先端大)
Yousoo Kim (RIKEN)/金有洙(理研)
Tetsuo Hanaguri (RIKEN)/花栗哲郎(理研)
Yukio Hasegawa (Univ. Tokyo)/長谷川幸雄(東京大)
Masahiro Haze (Univ. Tokyo)/土師将裕(東京大)
Yoshinori Okada (OIST)/岡田佳憲(沖縄科技大)
Yoshiaki Sugimoto (Univ. Tokyo)/杉本宜昭(東京大)
Yasuo Yoshida (Kanazawa Univ.)/吉田靖雄(金沢大)
For further questions, please contact to Masahiro Haze (haze -at- issp.u-tokyo.ac.jp).
Please replace -at- to @.
お問い合わせは土師(haze -at- issp.u-tokyo.ac.jp)まで。
-at-を@に置き換えてください。